The ability to rapidly shrink down to bug size (and beyond) gives Ant-Man and the Wasp tremendous advantages. But it also comes with some scale-related drawbacks, most notably, more difficult breathing. Trick out their suits with insect-inspired microscale air pumps, compressors, and molecule filters, combined with the fictional "Pym particle" technology, et voila! Problem solved.
Anne Staples, a bioengineer at Virginia Tech, and her graduate student Max Mikel-Stites first outlined the respiratory difficulties Ant-Man and the Wasp would likely face while insect-sized in a paper published this summer in the fledgling journal Superhero Science and Technology. (Can I just say how delighted I am that this journal exists?) The group researches respiration at the microscale, using insects as models. They described their work at a meeting of the American Physical Society's Division of Fluid Dynamics in Atlanta, Georgia.
Mikel-Stites, a fan of the Marvel cinematic universe, was stoked for Ant-Man and the Wasp's release. So one day in the lab last spring, the conversation naturally turned to how difficult it would be for the superheroes to breathe when insect-sized. "Applying that perspective to Ant-Man and the Wasp seemed like a straightforward thing to do," says Mikel-Stites, who admits to being a bit nitpicky when it comes to science in the movies. And he couldn't stop thinking about the breathing problems that our superheroes would inevitably face.
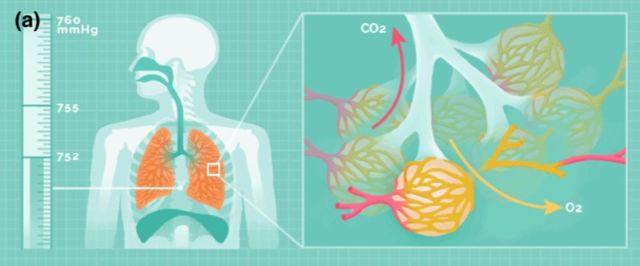
The smaller the animal, the less metabolically efficient it is, according to Kleiber's law (named after biologist Max Kleiber). That's probably because the surface area-to-volume ratio increases as objects get smaller. "Animals create heat in amounts proportional to their body's volume, but dissipate heat in amounts proportional to their body's surface area," Staples explains. "So small animals, which have high surface area-to-volume ratios, dissipate heat at high rates and cant stay warm.” To compensate for the heat loss, they need higher metabolic rates. Small animals therefore produce more heat and require more oxygen than larger ones.
Granted, it's not clear from the Marvel films if Ant-Man's mass also scales down when he shrinks. "Sometimes it appears he has the mass of a human—he falls and cracks a tile floor—and sometimes he appears to have the mass of an ant [when] he runs on the barrel of a gun and rides on Ant-thony, his ant friend," says Staples.
"The subjective atmospheric density experienced by a human who shrinks down to insect size changes."
They decided to assume that the masses of Ant-Man and the Wasp scaled down by eight orders of magnitude when they shrank down to insect size. That means their total metabolic rates would only scale down by six orders of magnitude. This translates into a per-unit mass 100 times greater than the superheroes would have when they are human-sized, so they would need 100 times more oxygen to function.
"While the actual atmospheric density is the same for an insect and a human, the subjective atmospheric density experienced by a human who shrinks down to insect size changes," says Mikel-Stites. When Scott Lang inhales at his normal size, he breathes in a certain number of oxygen molecules. Shrink down to ant-size, however, and he still needs the same number of oxygen molecules, but collects far less with each breath.
It's equivalent to what mountain climbers on Mt. Everest experience in the summit's infamous "death zone" at 7,998 meters above sea level. Most people respond to these conditions by breathing more rapidly to bring in more oxygen, if only to avoid the headache and dizziness common to altitude sickness.
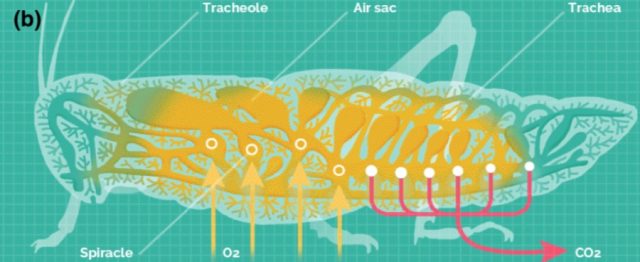
Fortunately microfluidic devices—the kind Staples and her group develop—could help. Insects and humans evolved very different breathing strategies due to the vastly different scales at which they live. According to Staples, many insects collapse their tracheal pathways when they breathe. No two insects do this in exactly the same way, but it usually involves abdominal contractions to trigger the collapse. "Sometimes the collapses propagate along the tracheal pathways in a contraction wave, and sometimes the collapses happen at separate locations along the same tracheal pathway," she says.
The Virginia Tech team's devices mimic different combinations of these three key features of insect breathing. By exploiting those strategies, the group has managed to build four (so far) insect-inspired tiny lab-on-a-chip machines that allow them to control fluids at small scales with great precision, with no need for pesky valves. A paper on this work is pending publication, and Staples' colleague, Krishnashis Chatterjee, described some preliminary results at the conference.
So, how could microfluidics help our superhero friends breathe at smaller size scales? To make up for the insufficient suction force to draw air into the helmet's mask, it would be possible to pump the air in with something called a "Knudsen pump." This relies on differences in temperature to pump gases (like air) through nanoscale pores in many minerals. There would definitely be a temperature difference inside and outside the Ant-Man and Wasp suits.
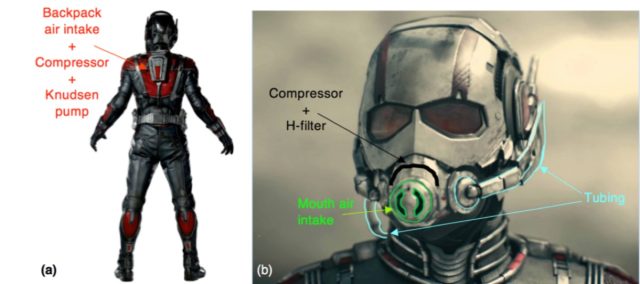
Next, there would have to be some means of compressing the air supply to achieve oxygen molecule densities on par with sea level (as opposed to Everest's high-altitude death zone). This assumes Ant-Man and the Wasp retain their human masses. "Compressing the air would allow them to get the same number of oxygen molecules in one lungful of air," Staples explains. "There are a number of microscale compressor technologies available, such as microscale diaphragm compressors, which can be operated mechanically or electrostatically."
Finally, adding a molecular filter (like an H-filter) could also help the superheroes cope with the increased oxygen demands. Such a filter would remove smaller non-oxygen molecules from the air, increasing the relative oxygen content, by exploiting the different diffusion time scales for differently sized molecules. Combine these three with Pym particles—said to allow for a reduction or expansion in the distance between atoms and matter, as well as manipulating mass—and you've got a viable solution to the breathing issue.
Staples' group debated which superhero they should choose for their next foray into comic book physics. Ever the Marvel fan, Mikel-Stites pushed for Dazzler, who has sonoluminescent powers (think the sonic shock wave produced by the powerful snap of a mantis shrimp's claw, writ large). But undergraduate student Afreen Khoja (and DC Comics) won out: they'll be investigating the hydrokinetic powers of Mera, Aquaman's Princess of Atlantis.
DOI: Superhero Science and Technology, 2018. 10.24413/sst.2018.1.2474 (About DOIs).
[contf] [contfnew]
Ars Technica
[contfnewc] [contfnewc]