Isaac Asimov dubbed neutrinos "ghost particles." John Updike immortalized them in verse. They've been the subject of several Nobel Prize citations, because these weird tiny particles just keep surprising physicists. And now we have a much better idea of the upper limit of what their rest mass could be, thanks to the first results from the Karlsruhe Tritium Neutrino experiment (KATRIN) in Germany. Leaders from the experiment announced their results last week at a scientific conference in Japan and posted a preprint to the physics arXiv.
"Knowing the mass of the neutrino will allow scientists to answer fundamental questions in cosmology, astrophysics, and particle physics, such as how the universe evolved or what physics exists beyond the Standard Model," said Hamish Robertson, a KATRIN scientist and professor emeritus of physics at the University of Washington. "These findings by the KATRIN collaboration reduce the previous mass range for the neutrino by a factor of two, place more stringent criteria on what the neutrino's mass actually is, and provide a path forward to measure its value definitively."
The ghostly particles are devilishly hard to detect because they so rarely interact with other particles, and when they do, they only interact via the weak nuclear force. Most neutrino hunters bury their experiments deep underground, the better to cancel out noisy interference from other sources, notably the cosmic rays continually bombarding Earth's atmosphere. The experiments usually require enormous tanks of liquid—dry-cleaning fluid, water, heavy water, mineral oil, chlorine, or gallium, for example, depending on the experimental setup. This increases the chances of a neutrino striking one of the atoms in the medium of choice, triggering the decay process. The atom changes into a different element, emitting an electron in the process, which can be detected.
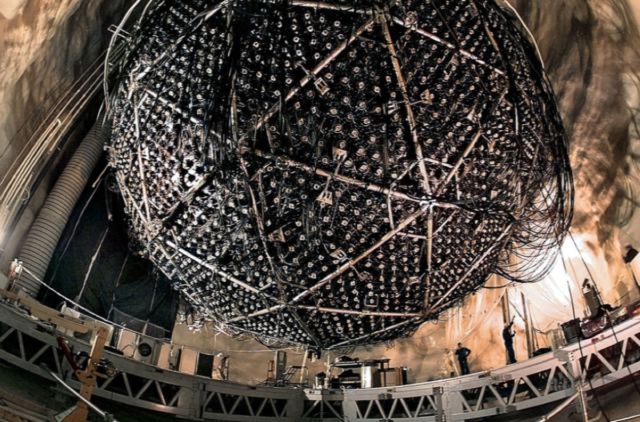
Neutrinos were first proposed by Wolfgang Pauli in a 1930 letter to colleagues. He was trying to explain some baffling experimental results on radioactive beta decay in atomic nuclei, where energy appeared to be missing—something he deemed (correctly) to be impossible. He thought a new kind of subatomic particle with no charge and no mass may have carried away the missing energy; it was Enrico Fermi who later dubbed it a neutrino.
Clyde Cowan and Frederick Reines were the first to observe these ghostly particles in 1956, thanks to the fusion reactions in nuclear power plants that proliferated after World War II. Ten years later, physicists detected the first solar neutrinos from the Sun. This snagged Ray Davis Jr. and Masatoshi Koshiba a Nobel Prize in 2002, shared with Riccardo Giacconi (who was honored "for pioneering contributions to astrophysics, which have led to the discovery of cosmic X-ray sources").
The only problem was that there were far fewer solar neutrinos being detected than predicted by theory, a conundrum that became known as the solar neutrino problem. In 1962, physicists discovered a second type ("flavor") of neutrino, the muon neutrino. This was followed by the discovery of a third flavor, the tau neutrino, in 2000.
By then, physicists already suspected that neutrinos might be able to switch from one flavor to another, thanks in large part to 1998 observations by Japan's Super-Kamiokande collaboration (Super-K). In 2002, scientists at the Sudbury Neutrino Observatory (or SNO) announced they had solved the solar neutrino problem. The missing solar (electron) neutrinos were just in disguise, having changed into a different flavor on the long journey between the Sun and the Earth. Arthur B. McDonald of SNO and Takaaki Kajita of Super-K shared the 2015 Nobel Prize in Physics for their respective breakthroughs.
If neutrinos oscillate, then they must have a teensy bit of mass after all. As Adrian Cho explained in a 2016 article for Science, "Were neutrinos massless, they would have to move at light speed, at least in a vacuum, according to Einstein's theory of relativity. If that were the case, time for them would stand still, and change would be impossible."
But determining precisely what that mass is constitutes another knotty neutrino-related problem. There are three neutrino flavors, but none of them has a well-defined mass. Rather, different kinds of "mass states" mix together in various ways to produce electron, muon, and tau neutrinos. That's quantum weirdness for you.
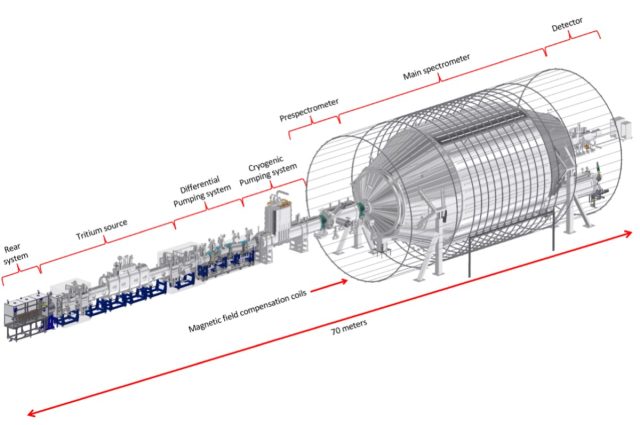