There are many insects that boast one or two bright colors on their cells. But the so-called "rainbow weevil" is unique because it has many different colored spots. Now researchers from Yale-NUS College and the University of Fribourg in Switzerland have discovered the mechanism behind this rainbow effect, and it is very like the way that squid or cuttlefish shift color for camouflage. They described their results in a recent paper in the journal Small.
Nature produces color in its creatures in various ways. For instance, the bright colors in butterfly wings don't come from any pigment molecules but from how the wings are structured. The scales of chitin (a polysaccharide common to insects) are arranged like roof tiles. Essentially they form a diffraction grating, except photonic crystals only produce certain colors, or wavelengths, of light, while a diffraction grating will produce the entire spectrum, much like a prism.
This is a naturally occurring example of what physicists call photonic crystals, or photonic bandgap materials. That's because photonic crystals are "tunable," precisely ordered in such a way as to block certain wavelengths of light while letting others through. Alter the structure by changing the size of the tiles, and the crystals become sensitive to a different wavelength. Even better (from an applications standpoint), the perception of color doesn't depend on the viewing angle.
Bags of pigment
Squid, cuttlefish, and octopodes get their shifting iridescent colors in a bit more complicated fashion. They have special pigment cells called chromatophores lining the outer later of their skin, each attached to muscle fibers which are, in turn, attached to a nerve fiber. Electrical pulses travel along the nerve to the muscles, which can expand and contract in response. If, for instance, the black cells shrink (contract), the pigmented area also shrinks and the animal's color will lighten. If all the red chromatophores expand, it will flush bright red.
But beneath the chromatophores is a separate layer of cells called iridophores. They are similar to the scales in butterfly wings in that their color is not pigment based, but structural. They are also tunable to different wavelengths of light, apparently via a neurotransmitter (a brain chemical called acetylcholine). It's a slower process to change those colors than to expand or contract chromatophores, but it can be done. In fact, back in 2012, a group called the Backyard Brains ingeniously connected an iPhone to the dorsal fins of longfin inshore squid to simulate the electrical signals of nerve cells, essentially turning the cells into actuators to convert sound into electrical impulses. The voltage changes in turn produced motion in the squid's skin cells in response to beat of Cypress Hills' "Insane in the Membrane." (You can watch the video here.)
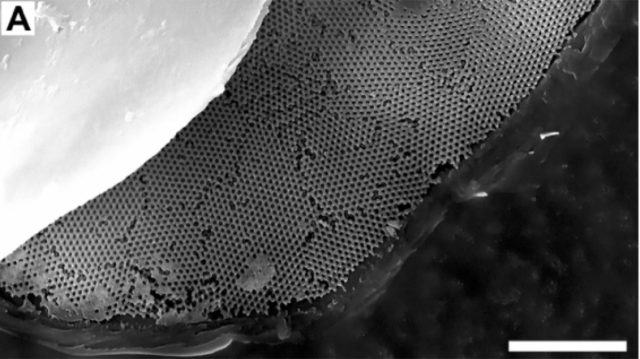
The rainbow weevil appears to use a similar mechanism to the squid, cuttlefish, and octopus. The researchers put the creatures under a scanning electron microscope and used high-energy X-ray imaging, to get a clearer picture of the underlying structure. The weevil's spots consist of circular scales of chitin, neatly arranged into concentric rings of varying hues like a rainbow: blue in the center, all the way out to red at the edges. The weevil's colors are determined both by the size of the crystal structure of each individual scale and also by how much chitin is used to build it. Red corresponds to larger scales and more chitin, while blue results from smaller scales and less chitin.
This is quite unusual: size and volume of chitin are usually fixed in animals who employ a similar color-generating mechanism. Somehow this insect can control both the size of its scales and how much chitin is used to fine-tune those colors as needed. Ultimately, the team hopes to determine exactly how the weevil achieves such precisely ordered structures, since it's not yet possible to produce photonic crystals at this size scale in the laboratory—although there are plenty of other types of manmade photonics crystals. Their tunability makes them ideal for a wide range of applications.
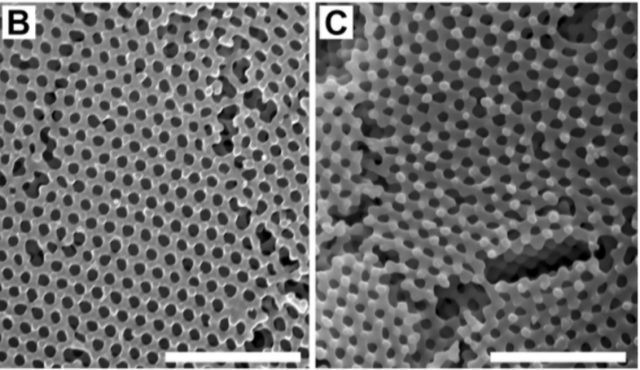
"We can use these structures in cosmetics and other pigmentations to ensure high-fidelity hues or in digital displays in your phone or tablet, which will allow you to view it from any angle and see the same true image without any color distortion," says co-author Vinodkumar Saranathan. "We can even use them to make reflective cladding for optical fibers to minimize signal loss during transmission."
DOI: Small, 2018. 10.1002/smll.201802328 (About DOIs).
[contf] [contfnew]
Ars Technica
[contfnewc] [contfnewc]